Why do water molecules stick to the surface?
Think of it like this: Imagine a bunch of tiny magnets, each with a positive and negative side. When these magnets are close together, the positive side of one magnet will be attracted to the negative side of another magnet. Water molecules are like these magnets, sticking to each other through hydrogen bonds.
This cohesion is what makes water so unique. It’s why water droplets form and why water can flow in rivers and streams. It’s also why water has a high surface tension, allowing insects to walk on water. The cohesion of water is essential for life on Earth!
Why is there surface tension in water?
Imagine water molecules like tiny magnets, each with a positive and negative end. The positive end of one molecule is attracted to the negative end of another molecule. These attractions create a tight network, holding the molecules together.
This strong cohesive force is what makes water act like a thin, flexible skin. Think of a drop of water resting on a leaf. The surface tension makes the water bead up into a spherical shape, resisting the pull of gravity.
This surface tension also allows some insects to walk on water. The insects’ weight is distributed over a large enough area, and the strong surface tension of water is able to support their weight.
The strength of surface tension depends on the temperature. As the temperature increases, the cohesive forces between molecules weaken, and the surface tension decreases. That’s why it’s easier to break through the surface of warm water than cold water.
How does the molecular structure of water contribute to its high surface tension?
The hydrogen atom in one water molecule has a slight positive charge, while the oxygen atom carries a slight negative charge. This difference in charge creates an electrostatic attraction between the hydrogen atom of one water molecule and the oxygen atom of another. These special bonds are called hydrogen bonds.
Think of these hydrogen bonds like tiny magnets holding water molecules together. They create strong cohesive forces, pulling the water molecules close to each other. This strong attraction between water molecules gives water its high surface tension.
Surface tension is a property that allows a liquid to resist an external force, like a bug walking on the water’s surface. It’s like a thin, invisible skin stretched across the surface of the water. This skin is strong enough to support the weight of small objects, like a tiny insect.
The hydrogen bonds between water molecules create a network that is hard to break. This network is what gives water its unique properties.
Imagine a water droplet. It’s a sphere because the water molecules at the surface are pulled inwards by the cohesive forces of the hydrogen bonds. These bonds create a balance between the inward pull of the water molecules and the outward force of gravity.
Let’s consider another example. Imagine a water strider bug. It can walk on water because its legs are long and slender, distributing its weight evenly across the water’s surface. The surface tension of the water provides enough support to hold the bug up.
So, the next time you see a water strider bug gracefully gliding across the water, remember it’s the powerful hydrogen bonds that are keeping it afloat!
Why doesn’t oil mix with water mastering biology?
Water is a polar molecule, meaning it has a positive and negative end. This is because the oxygen atom in water pulls the electrons closer to it, making the oxygen side slightly negative and the hydrogen side slightly positive. These opposite charges create hydrogen bonds between water molecules, which are strong attractions.
Oils and fats, on the other hand, are nonpolar. They don’t have positive or negative ends, so they don’t form hydrogen bonds with water. In fact, water molecules are so strongly attracted to each other that they push the nonpolar oil molecules away. This is why oil and water separate into distinct layers.
Think of it like this: imagine you have a group of people holding hands tightly in a circle. These are the water molecules, linked by hydrogen bonds. Now, try to push a group of people who aren’t holding hands (the oil molecules) into the circle. The people holding hands will naturally resist and keep the other group out!
You can also think about it in terms of energy. For oil to dissolve in water, it would need to break some of the water’s hydrogen bonds. This requires a lot of energy, and it’s not energetically favorable. It’s much easier for the oil molecules to stay together, forming their own separate layer.
Here’s another way to look at it: Imagine a group of people at a party. The water molecules are all chatting and laughing together, forming strong bonds (like hydrogen bonds). Now, imagine a group of people who only speak a different language. They try to join the party, but they can’t understand anyone and are unable to connect with the group. They end up staying separate because they don’t have anything in common. This is like oil and water. Oil and water molecules simply don’t “speak the same language” and are unable to form bonds with each other.
Why do hydrophobic molecules stick together?
Think of it like this: oil and water don’t mix. Oil is hydrophobic, meaning it doesn’t like water. When you mix oil and water, the oil molecules clump together to avoid the water. This is because the water molecules are attracted to each other and form hydrogen bonds, pushing the oil molecules away.
This is how fat molecules are able to travel through our bodies. Fat molecules are hydrophobic, so they travel through our bloodstream in small packages called lipoproteins. The outer layer of a lipoprotein is made of phospholipids, which are amphipathic. This means that one part of the phospholipid is hydrophilic (likes water) and the other part is hydrophobic (doesn’t like water). This allows the lipoprotein to carry the fat molecules through the watery environment of our blood.
The hydrophobic parts of the phospholipids cluster together in the center of the lipoprotein, keeping the fat molecules safe and protected. The hydrophilic parts of the phospholipids face outward, allowing the lipoprotein to move freely through the water-based blood.
So, while hydrophobic molecules don’t like water, they do like to stick together! This is a very important process in our bodies, allowing us to transport and use fat for energy and other important functions.
What causes molecules to stick together in liquid water?
The partial positive charge on the hydrogen atom of one water molecule is attracted to the partial negative charge on the oxygen atom of another water molecule. This attraction is what forms the hydrogen bond. These bonds are relatively weak, but they are numerous and they play a crucial role in the properties of water.
Think of it like magnets. The opposite poles of two magnets attract each other. The same thing happens with water molecules, but instead of magnetic poles, we have partial positive and negative charges.
Let’s dive a little deeper into why this matters. Water’s unique properties, like its high boiling point and its ability to dissolve many substances, are largely due to these hydrogen bonds. The hydrogen bonds create a strong attraction between water molecules, which requires a significant amount of energy to break. This is why water has a relatively high boiling point.
Without these bonds, water would likely be a gas at room temperature. Imagine a world where water evaporated instantly!
Think about this, the hydrogen bonds between water molecules are what make liquid water, liquid. The bonds constantly break and reform, allowing the molecules to move around but remain close together.
Why does surface tension exist at the surface?
Imagine a liquid like water. Inside the water, each molecule is surrounded by other water molecules, pulling on it equally from all directions. This creates a balanced situation where the forces cancel each other out. However, at the surface, things are different.
The molecules at the surface have fewer neighbors above them compared to those below them. They experience an inward pull from the molecules below, but not an equal pull from above. This imbalance creates a net inward force, pulling the surface molecules together.
This inward force is what creates surface tension. The molecules at the surface tend to minimize their energy by decreasing the surface area. Think of it like a stretched rubber band. It wants to relax and contract, and the surface of a liquid behaves in a similar way. This minimization of surface area is why water droplets form into spheres, as spheres have the smallest surface area for a given volume.
Let’s dive deeper into the forces at play:
The forces between molecules that cause surface tension are known as cohesive forces. These forces are attractive forces that hold the molecules together. Cohesive forces are responsible for the properties of liquids, such as their viscosity and surface tension. They arise from various types of interactions, including hydrogen bonding, Van der Waals forces, and dipole-dipole interactions.
The strength of these cohesive forces directly affects the surface tension of a liquid. Liquids with strong cohesive forces, like water, have high surface tension. Liquids with weaker cohesive forces, like alcohol, have lower surface tension.
The concept of surface tension plays a crucial role in understanding many natural phenomena. It helps explain why insects can walk on water, how raindrops form, and why soap bubbles exist. It is a fundamental concept in physics and chemistry that provides valuable insights into the behavior of matter at the molecular level.
What is the molecular theory of surface tension?
Imagine a liquid like water, with its molecules constantly moving and interacting. At the liquid-gas interface, things are a bit different. The molecules at the surface feel different forces compared to those deep inside the liquid.
Think of it like this:
Inside the liquid: Molecules are surrounded by similar molecules on all sides, so they experience attractive forces pulling them equally in all directions.
At the surface: Molecules only have attractive forces from the liquid below, not from the gas above. This creates an imbalance.
This imbalance leads to surface tension, which is like a thin, invisible skin pulling the liquid’s surface inwards. This tension is what allows a water strider to walk on water, or a drop of water to form a spherical shape.
Now, how does this relate to the Helmholtz free energy? This is a thermodynamic concept that helps us understand the stability of systems. In the van der Waals approach, we use the calculus of variations to find the minimum Helmholtz free energy for a system. This minimum represents the most stable state.
In our case, the Helmholtz free energy of the liquid-gas interface is affected by the density of the liquid. We can use a mathematical expansion to express this energy as a function of the density’s derivatives. By minimizing this expression, we can find the equilibrium density profile for the liquid at the surface.
This equilibrium profile is the one that leads to the surface tension we observe. The calculus of variations is a powerful tool that allows us to calculate this profile and, ultimately, understand the molecular basis of surface tension.
See more here: Why Is There Surface Tension In Water? | Why Do Amphipathic Molecules Line Up At A Water Surface
How do amphipathic molecules form a stable membrane?
Imagine you have a bunch of these phospholipids in water. The hydrophilic heads are drawn to the water molecules and happily mingle with them. However, the hydrophobic tails don’t want anything to do with water. They try to avoid it at all costs. So, what happens? The phospholipids gather together, with their hydrophobic tails pointing inwards, away from the water, and their hydrophilic heads facing outwards, towards the water.
This arrangement creates a double layer, with two rows of phospholipids facing each other, forming a lipid bilayer. The hydrophobic tails form the inner core of the membrane, shielded from the watery environment, while the hydrophilic heads interact with the water on either side of the membrane. This creates a stable barrier that separates the inside of a cell from its external environment.
This lipid bilayer isn’t just a static wall; it’s a dynamic and flexible structure. It can allow certain molecules to pass through while blocking others, acting as a selective gatekeeper for the cell. This controlled movement of molecules is essential for the cell’s survival and function. It allows nutrients to enter, waste products to exit, and signals to be transmitted.
So, thanks to the unique properties of amphipathic molecules like phospholipids, cells can create the stable membranes that allow them to exist and thrive. This seemingly simple arrangement of molecules is a cornerstone of life, showcasing the elegance and efficiency of nature’s design.
Why is amphipathy important?
Imagine a group of amphipathic molecules thrown into a pool of water. The hydrophilic heads, eager to mingle with water, will readily dive in. However, the hydrophobic tails, shunning the water, will try to avoid it. This push and pull creates a unique arrangement where the hydrophilic heads face the water while the hydrophobic tails cluster together, forming a barrier between the watery environment and the cell’s interior.
This self-assembly process is fundamental to the formation of biological membranes. These membranes act as protective barriers around cells, controlling what goes in and out. Without amphipathy, cells wouldn’t be able to maintain their structure or function.
But the importance of amphipathy extends beyond cell membranes. Proteins, the workhorses of our bodies, also rely on amphipathy. Some proteins have amphipathic regions that allow them to interact with both water and lipids, enabling them to function within the complex environment of a cell.
Let’s take a closer look at the formation of cell membranes. Amphipathic phospholipids, the main building blocks of membranes, consist of a hydrophilic head and two hydrophobic tails. When these molecules are placed in water, they spontaneously assemble into a bilayer, where the hydrophilic heads face outward towards the watery environment, and the hydrophobic tails are tucked away inside the bilayer, shielded from the water. This bilayer forms a stable and flexible barrier that encloses the cell and regulates the movement of molecules across the membrane.
The ability of amphipathic molecules to form these self-assembled structures is crucial for life. It allows cells to maintain their structure and function, regulate their internal environment, and interact with their surroundings. This is why we can say that amphipathy is indeed fundamental to the structure of life.
How do amphipathic molecules assemble?
In water, amphipathic molecules spontaneously form structures called micelles. Micelles are tiny spheres where the hydrophobic tails of the molecules cluster together in the center, away from the water, while the hydrophilic heads face outward, interacting with the surrounding water molecules. This arrangement minimizes the contact between the hydrophobic tails and the water, leading to a stable structure.
The formation of micelles is driven by a few key factors. First, the hydrophobic effect pushes the hydrophobic tails away from the water, forcing them to cluster together. Second, the hydrophilic heads, attracted to the water, arrange themselves on the outside of the micelle, creating a barrier between the water and the hydrophobic core. The result is a stable structure that allows amphipathic molecules to exist in aqueous environments.
The formation of micelles is a fascinating example of how the structure of molecules influences their behavior. This property of amphipathic molecules is crucial in many biological processes, including the transport of fats and other lipids, and the formation of cell membranes.
Are phospholipids amphipathic or hydrophilic?
Phospholipids are like little “two-faced” molecules, meaning they have both a hydrophilic (water-loving) head and a hydrophobic (water-fearing) tail. Think of it this way: the head is like a friendly extrovert that loves to hang out with water molecules, while the tail is a shy introvert that prefers to avoid water at all costs.
The hydrophilic head of a phospholipid is made up of a phosphate molecule and a glycerol molecule. These molecules are polar, meaning they have an uneven distribution of electrical charge. This polarity allows them to interact easily with water molecules, which are also polar.
The hydrophobic tail is composed of fatty acids. These are long chains of hydrocarbons, which are nonpolar. Because they lack a charge, fatty acids don’t mix well with water.
So, how does this “two-faced” nature of phospholipids play out in the real world? Well, phospholipids are the building blocks of biological membranes, like the cell membrane that surrounds every cell in your body. These membranes act as barriers, controlling what goes in and out of cells.
Here’s where the amphipathic nature of phospholipids comes in: the hydrophilic heads of the phospholipids face outward, towards the watery environment inside and outside the cell, while the hydrophobic tails face inwards, forming a barrier that keeps water out. This arrangement creates a stable, semi-permeable membrane that protects the cell and allows for controlled transport of molecules.
It’s like a team of introverted fatty acids holding hands in the middle, surrounded by a circle of friendly phosphate groups, ready to greet the water molecules on either side! This arrangement is what makes biological membranes possible, and it’s a testament to the amazing design of the natural world.
See more new information: linksofstrathaven.com
Why Do Amphipathic Molecules Line Up At A Water Surface?
Have you ever wondered why soap bubbles form, or why oil and water don’t mix? The answer lies in the unique properties of amphipathic molecules, molecules with both hydrophilic (water-loving) and hydrophobic (water-fearing) parts. These molecules, like little two-faced characters, play a starring role in the fascinating world of surface tension and how liquids behave.
Imagine a water surface. It’s like a tiny, invisible trampoline, holding the water molecules together. The water molecules are attracted to each other by hydrogen bonds, creating a strong “skin” on the surface. But this “skin” isn’t always happy. It’s constantly trying to minimize its surface area, which is why water droplets form spheres.
Now, enter the amphipathic molecule. It’s got a hydrophilic head that’s drawn to the water, and a hydrophobic tail that desperately wants to avoid the water. When you add these molecules to water, they’ll do whatever it takes to feel comfortable. The hydrophilic head dives into the water, while the hydrophobic tail tries to escape, pushing itself out of the water and into the air.
This creates a fascinating situation. The amphipathic molecules line up at the water surface, their heads happily swimming in the water, and their tails poking out into the air. It’s like a tiny, organized army, standing shoulder-to-shoulder, creating a barrier between the water and the air. This barrier is called a monolayer.
Think of it like a line of people holding hands. The people with their hands outstretched are the hydrophilic heads, happily in the water. The people with their arms crossed, facing away from the water, are the hydrophobic tails, avoiding the water.
This lining up at the surface isn’t just a neat trick. It actually reduces the surface tension of the water. Remember that invisible trampoline? The amphipathic molecules weaken its strength, making the water less resistant to stretching.
Think about how soap makes it easier to wash your hands. The soap molecules are amphipathic, and they line up at the surface of water. They help to break the surface tension, allowing the water to mix more easily with the dirt and grime on your hands.
The same principle applies to how detergents clean clothes. They also contain amphipathic molecules that disrupt the surface tension of water, allowing it to penetrate the fabric and lift away dirt and grease.
But amphipathic molecules are not just about cleaning. They play a crucial role in biological membranes. Think of the cell membrane that surrounds every living cell. It’s made of a double layer of phospholipids, which are amphipathic molecules. Their hydrophilic heads face the watery environment inside and outside the cell, while their hydrophobic tails hide within the membrane, creating a barrier that protects the cell from its surroundings.
The importance of amphipathic molecules can’t be overstated. They’re essential for life, cleaning, and even the formation of bubbles. Their ability to line up at water surfaces is a testament to their fascinating duality and their incredible influence on the world around us.
FAQs about Amphipathic Molecules at the Water Surface:
1. Why do amphipathic molecules only line up at the water surface?
Amphipathic molecules line up at the water surface because they want to find the most comfortable position. They can’t fully dissolve in water because their hydrophobic tails are repelled by the water molecules. They also can’t fully dissolve in air because their hydrophilic heads need to be surrounded by water. The surface provides the perfect compromise, allowing the hydrophilic heads to be in contact with water and the hydrophobic tails to be in contact with air.
2. Can amphipathic molecules line up at other surfaces?
Yes, amphipathic molecules can line up at other surfaces, like the surface of oil or a solid material. The specific arrangement will depend on the nature of the surface and the amphipathic molecule itself.
3. What are some examples of amphipathic molecules besides soap?
Besides soap, some other examples of amphipathic molecules include:
– Phospholipids: These are the main component of cell membranes.
– Detergents: These are used in cleaning products to remove dirt and grease.
– Emulsifiers: These help to mix oil and water, for example, in mayonnaise or salad dressings.
– Surfactants: These are used in a variety of applications, including in shampoos, cosmetics, and food products.
4. How do amphipathic molecules affect the surface tension of water?
Amphipathic molecules reduce the surface tension of water. They do this by disrupting the hydrogen bonds between water molecules at the surface. This makes the water less resistant to stretching and allows for easier mixing with other substances.
5. Is the lining up of amphipathic molecules a spontaneous process?
Yes, the lining up of amphipathic molecules at the water surface is a spontaneous process driven by the hydrophobic effect. The hydrophobic tails want to minimize their contact with water, and this is achieved by aligning themselves at the surface.
6. Do amphipathic molecules always form a monolayer at the water surface?
While amphipathic molecules typically form a monolayer at the water surface, the exact arrangement can vary depending on the concentration of the amphipathic molecules, the temperature, and other factors. At higher concentrations, they may form multiple layers, or even micelles, which are spherical structures with the hydrophobic tails pointing inwards and the hydrophilic heads pointing outwards.
7. What are micelles?
Micelles are spherical structures formed by amphipathic molecules in solution. The hydrophobic tails of the molecules point inwards, forming a core that is shielded from the water. The hydrophilic heads point outwards, interacting with the surrounding water molecules. Micelles are important in many biological and industrial processes, including the digestion of fats, the transportation of nutrients, and the formation of detergents.
Understanding the behavior of amphipathic molecules helps us understand the world around us, from how soap cleans to how our cells function. They’re tiny but mighty, playing a key role in a vast range of processes.
Biology Chapter 3- Mastering Questions Flashcards | Quizlet
Which statement must be mentioned in explaining why amphipathic molecules line up at a water surface? Polar groups attract one another. Dissolving is best described as … Quizlet
Mastering Biology – Properties of water Flashcards | Quizlet
Life realist on the properties of water. Water organizes other kinds of molecules into structures and make up cells. Water organizes molecules on the basis of their polarity. Quizlet
Amphipathic – Definition & Examples of Amphipathic
Phospholipids – the type of amphipathic molecule that makes up most cell membranes – are able to form a stable membrane because their “head” is attracted to water molecules, while their Biology Dictionary
Chapter 2 Lab – Mastering Flashcards | Quizlet
Study with Quizlet and memorize flashcards containing terms like Which statement must be mentioned in explaining why amphipathic molecules line up at a water surface? Quizlet
11.7: Amphipathic Lipids and Membranes
The ionic part is referred to as hydrophilic, meaning “water loving,” and the nonpolar part as hydrophobic, meaning “water fearing” (repelled by water). The amphipathic lipids commonly found in membranes are Chemistry LibreTexts
Amphipathic Molecules | Definition & Examples – Lesson
An amphipathic molecule is a compound comprising a hydrophilic head and a hydrophobic tail. The hydrophilic head is the polar part interacting with water, while the hydrophobic tail is the… Study.com
Organizing Effects of Water – pearsoncmg.com
Water organizes other kinds of molecules into structures that make up cells. Water organizes molecules on the basis of their polarity. In this respect, molecules fall into pearsoncmg.com
Amphiphile – Wikipedia
An amphiphile (from the Greek αμφις amphis, both, and φιλíα philia, love, friendship), or amphipath, is a chemical compound possessing both hydrophilic (water-loving, polar) Wikipedia
4.6: Non-Membrane Lipid Assemblies (Micelles) – Physics
Its amphipathic properties allow detergents to dissolve both water and oil soluble compounds, such as soap 2. In soap, particles insoluble in water (oil/fat) become Physics LibreTexts
Properties Of Water
How Polarity Makes Water Behave Strangely – Christina Kleinberg
Topic 2.7 – Amphiphilic Molecules
Amphipathic Molecules In Biology
Why Is Water Polar? Why Does Water Have A Bent Shape?
3D Molecular Visualisation – Water Turning Into Ice
Amphiphiles – Soaps, Detergents, Phospholipids, Surfactants, Etc.
Link to this article: why do amphipathic molecules line up at a water surface.
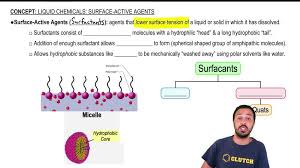
See more articles in the same category here: https://linksofstrathaven.com/how