What is the lifetime of an electron in the excited state?
Understanding Excited States and Lifetimes
Imagine an electron in an atom like a ball on a staircase. The electron can occupy different energy levels, represented by the steps of the staircase. The ground state is the lowest energy level, like the bottom step. When an electron absorbs energy, it jumps to a higher energy level, like climbing up the staircase. This is called an excited state.
However, excited states are unstable. The electron wants to return to its ground state, releasing the extra energy it gained. This release can happen through various processes, such as emitting light (photons) or transferring energy to other atoms or molecules. The lifetime of an excited state refers to how long, on average, it takes for the electron to return to its ground state.
For a hydrogen atom, the lifetime of an excited state is typically on the order of 10-8 seconds. This short lifetime reflects the fact that electrons in excited states are eager to lose their excess energy and return to a more stable state.
Factors Affecting Lifetime
The lifetime of an excited state can be influenced by several factors, including:
The specific energy level: Higher energy levels tend to have shorter lifetimes because the electron has more energy to lose.
The atom’s environment: Collisions with other atoms or molecules can influence the rate of energy release.
The presence of external fields: Electric and magnetic fields can affect the energy levels and lifetimes of excited states.
Implications of Excited State Lifetimes
Understanding the lifetimes of excited states is crucial in various fields:
Spectroscopy: The wavelengths of emitted light can be used to identify the elements present in a sample.
Lasers: The principle of stimulated emission, where excited atoms are encouraged to emit light together, forms the basis of lasers.
Chemical reactions: Energy transfer processes involving excited states play a significant role in many chemical reactions.
The study of excited state lifetimes provides valuable insights into the behavior of atoms and molecules, with implications for diverse scientific and technological applications.
Do metastable states have a larger lifetime than excited states?
Here’s what makes metastable states so interesting: They’re kind of like a balancing act. To get from a metastable state to the ground state, you need a little push, or a nudge. It’s not like a regular excited state, which wants to jump back down to the ground state as quickly as possible. You could even imagine a metastable state as a little hilltop on a landscape. The ball at the top of the hill is in a metastable state. It’s not going to roll down to the bottom (ground state) on its own. It needs a little push or a bump to get going.
Metastable states are everywhere in science, from lasers to nuclear reactions. They are essential for many important technologies, such as lasers and semiconductor devices. For example, lasers use metastable states to create a population inversion, a condition where more atoms are in an excited state than in the ground state. This allows the laser to amplify light and produce a coherent beam.
So, to answer your question: Yes, metastable states have a longer lifetime than excited states. But they don’t last forever. Eventually, they’ll make their way back to the ground state.
What is the lifespan of an excited state?
However, it’s important to note that the lifetime of an excited state can vary significantly depending on several factors, including the specific molecule or atom, the environment surrounding it, and the nature of the excited state itself. For instance, some molecules can have excited states that last for microseconds or even milliseconds. This is because the decay process can be slowed down by various mechanisms, such as:
Non-radiative decay: In this process, the excited state loses energy through collisions with other molecules or by transferring energy to other parts of the molecule. This process is often more efficient than radiative decay, especially at high temperatures or in solution.
Intermolecular interactions: The presence of other molecules can influence the excited-state lifetime. For example, if a molecule is in a solvent, the solvent molecules can quench its excited state by interacting with it and causing it to lose energy.
Internal conversion: This process involves the molecule transitioning from a higher excited state to a lower one without emitting any light. This can happen if the energy difference between the two states is small.
Intersystem crossing: This process involves the excited state changing its spin state. This can occur when the excited state is a singlet state, and it transitions to a triplet state, which is a lower energy state but has a different spin.
Understanding excited-state lifetimes is crucial in various fields, including chemistry, physics, and biology. For example, in photochemistry, the lifetime of an excited state determines how long a molecule will remain in its reactive state and therefore its ability to undergo photochemical reactions. In fluorescence microscopy, the lifetime of an excited state is used to determine the properties of the molecules being studied.
What is the lifetime of the excited state in laser?
In reality, these lifetimes can range quite a bit. They might be as short as a picosecond, which is a thousand times faster than a nanosecond, or even linger for a few milliseconds, which is a thousand times longer than a nanosecond.
Now, what exactly does this lifetime mean, and why does it matter?
Imagine a laser atom like a tiny, energetic ball. When an atom absorbs energy, it jumps to a higher energy level, like our ball bouncing to a higher shelf. This is the excited state. But just like a ball doesn’t stay on the shelf forever, this excited atom wants to return to its normal, lower energy state. This “return trip” takes a certain amount of time – that’s the excited state lifetime.
A shorter lifetime means the atom quickly returns to its ground state. This is actually great for laser operation! A fast decay back to the ground state allows for more stimulated emission, leading to a stronger laser beam.
On the other hand, a longer lifetime means the excited atom spends more time in its higher energy state before returning to the ground. This can be useful for some applications, but it can also hinder laser operation if the excited state becomes too “populated” and less energy is available for stimulated emission.
Understanding the excited state lifetime is crucial to designing lasers with specific characteristics. It helps us optimize the laser’s power, efficiency, and even its pulse duration. So, the next time you see a laser, remember the tiny atoms buzzing with energy, and their fleeting yet crucial excited states!
What is the life span of electron in excited and metastable states?
Metastable states are like a special kind of excited state for an atom or molecule. Think of it like this: Imagine an atom is like a ball sitting at the bottom of a hill (the ground state). If you give it some energy, it can roll up the hill to a higher point (excited state). But there are some points on the hill that the ball might hang out for a bit longer than others, because it takes a little more energy to roll further down. Those are the metastable states.
The thing about metastable states is that they last longer than other excited states but still shorter than the ground state. How much longer? We’re talking about milliseconds, which might sound like a short time, but in the world of atoms, that’s a really long time. Imagine an atom in a metastable state holding its breath for a thousandth of a second.
Now, why are metastable states interesting? Well, because they can lead to some really cool phenomena, like lasers and fluorescence. Think of it like this: When an electron in a metastable state finally decides to come back down to its ground state, it releases that energy as light. This light can be used for all sorts of things, from reading bar codes to medical imaging.
So, how long *do* these electrons hang out in a metastable state? It depends on the atom or molecule. For most atoms, the lifetime of a metastable state is on the order of 10^-3 seconds (a millisecond), as you mentioned. That’s a very long time for an electron in an excited state! But it’s still much shorter than the ground state, which can be practically forever in atomic terms.
For some atoms, though, metastable states can last even longer, sometimes seconds or even minutes. This is because there are specific rules governing transitions between states that can make it much harder for an electron to jump down to a lower energy level. For instance, sometimes an electron might need to change its spin in order to make the transition, which can be very unlikely. So, those electrons can hang out in the metastable state for a much longer time.
It’s kind of like a traffic jam on the hill: Normally, the electrons can move down quickly, but sometimes there’s a bottleneck, and they get stuck in the metastable state for a while until things clear up. It might take a long time for them to find the right route down, but they eventually do, releasing their energy as light along the way.
How to find the lifetime of an electron?
Here’s how it works: Imagine you have a material with a lot of tiny pores, like a sponge. These pores act like tiny containers for electrons. When you apply a voltage to the material, electrons start to fill these pores. The time it takes for the voltage to rise tells us how quickly the electrons are filling the pores. This is the electron-transport time.
To calculate the electron-transport time, we need to know the capacity of the material. This capacity is a measure of how many electrons the pores can hold. We also need to know the capacity of the interface between the material and the electrolyte. The electrolyte is the solution that allows electrons to flow in and out of the material.
Once we have the electron-transport time and the capacity values, we can measure the voltage decay. This decay tells us how quickly the electrons are leaving the pores. The electron lifetime is the time it takes for half of the electrons to leave the pores. This gives us an idea of how long electrons stay in the material before they escape.
The electron lifetime is a crucial parameter for understanding how efficiently a material can store and release electrons. It is also important for understanding the performance of electronic devices based on these materials.
What is the distinction between excited state and metastable state?
Imagine an electron as a tiny ball in a valley. Its ground state is like being at the bottom of the valley, the most stable and lowest energy level. When an electron absorbs energy, it’s like giving that ball a push, making it climb higher up the valley walls. This higher position represents the excited state.
Now, a metastable state is like a small, flat plateau on the valley’s slope. It’s not as low as the ground state, but it’s not as high as some excited states. This means the electron is temporarily stuck, unable to immediately return to its ground state.
Think of it like this: when the electron gets excited, it might not immediately fall back down to the ground state. Instead, it could first settle into a metastable state. It’s like taking a detour on the way back home. This detour happens because the electron needs to release a specific amount of energy to go back to its ground state, and it may not have that energy available right away.
Essentially, the difference lies in the energy level and how long the electron stays in that state. An electron in an excited state is highly energetic and tends to quickly return to its ground state. On the other hand, an electron in a metastable state is less energetic, and it hangs around a bit longer before finally returning to its ground state.
What is the time period of a metastable state in a laser?
The lifetime of the upper laser level is how long an atom stays in that metastable state before it releases its energy. For some lasers, like erbium-doped fiber amplifiers, this can be quite a while – around 8 to 10 milliseconds. That’s a pretty long time for an atom to hang out in an excited state! For other lasers, like ytterbium-doped ones, the lifetime is a little shorter – around 1 to 2 milliseconds.
The lifetime of this metastable state is really important because it determines how much energy can be stored in the laser medium. The longer the lifetime, the more energy can be stored, and the more powerful the laser can be. This is why we’re so interested in metastable states!
Let me give you a little more detail about how these metastable states work. It all comes down to the energy levels of the atoms in the laser medium. When an atom absorbs energy, it jumps to a higher energy level. But this higher level isn’t always the highest possible level. Sometimes, the atom lands in a slightly lower energy level – this is called the metastable state.
This metastable state is kind of like a parking lot for the atom. It’s not the final destination, but it’s a place where the atom can hang out for a bit before it finally releases its energy. And this is where things get interesting.
You see, the metastable state is a special kind of energy level. It’s hard for the atom to drop back down to its ground state, so it stays in this metastable state for a longer time. It’s like the atom is holding its breath, waiting for the right moment to release its energy.
That’s what makes it so useful for lasers. Because the atoms are stuck in this metastable state for a while, it gives us time to build up a lot of energy in the laser medium. Then, when we trigger the laser, all those excited atoms release their energy at once, creating a powerful beam of light.
What is the lifetime of helium negative ion in metastable state?
The ~10 μs lifetime is associated with the 2s2p state of the ion. This state is relatively long-lived because it is forbidden to decay to the ground state by electric dipole transitions. However, it can decay through other mechanisms, such as magnetic dipole transitions or collisions with other particles.
The ~16 μs lifetime is associated with the 2s3s state of the ion. This state is also relatively long-lived, but it can decay to the ground state through electric dipole transitions. However, these transitions are relatively weak, leading to a longer lifetime.
The ~350 μs lifetime is associated with the 2s3p state of the ion. This state is the longest-lived of the three because it is also forbidden to decay to the ground state by electric dipole transitions. It can decay through other mechanisms, such as magnetic dipole transitions or collisions with other particles, but these processes are less likely, resulting in a significantly longer lifetime.
These lifetimes are important for understanding the behavior of helium negative ions in plasmas and other environments. The lifetimes influence how the ions interact with other particles, and they also determine the rate at which the ions decay to their ground state. This knowledge is vital for applications in areas like plasma physics, astrophysics, and even laser technology.
See more here: Do Metastable States Have A Larger Lifetime Than Excited States? | Lifetime Of Electron In Metastable State
What is a metastable atom?
Imagine an atom as a tiny, energetic house. Normally, the atom resides in its ground state, which is like the house being in its most stable, relaxed state. But sometimes, the atom gets a boost of energy – think of someone throwing a rock at the house, causing it to shake and vibrate. This is when the atom enters an excited state, which is like the house being temporarily energized and not in its usual, calm state.
Now, here’s where things get interesting. Usually, excited atoms quickly shed this extra energy and return to their ground state, like the house settling back down after the rock toss. However, there are some special excited states where the atom lingers for a surprisingly long time. This is called a metastable state. Think of this as the house vibrating for a long time, refusing to settle down right away.
Metastable states can last anywhere from a millionth of a second (10^-6 seconds) to a thousandth of a second (10^-3 seconds). That might not seem like a long time, but in the world of atoms, it’s a real stretch. These metastable states are like a pause button, allowing the atom to hold onto its energy for a while longer before eventually returning to its ground state.
Why do metastable states matter? They play a critical role in many scientific applications, from lasers to atomic clocks. These special states provide the foundation for technologies that shape our world.
Let’s dive a little deeper into why metastable atoms can hold onto their energy for longer periods. It all comes down to the rules of quantum mechanics, the physics that governs the behavior of atoms.
Imagine that when an excited atom wants to return to its ground state, it needs to take a specific set of “stairs” to get there. But, sometimes, there aren’t any convenient stairs to the ground floor. Instead, there might be a series of higher steps that need to be taken first. This means the atom must lose its energy in stages, which takes time.
Metastable states are like these “higher steps” in the quantum world. They represent energy levels that are difficult for the atom to transition out of. They are not the lowest energy state, but they are also not easily accessible to the atom, delaying its return to the ground state.
Think of it like this: Imagine a ball at the top of a hill. If there’s a smooth, clear path to the bottom, the ball will roll down quickly. But if there are bumps and obstacles on the path, the ball might get stuck on a small plateau before finally reaching the bottom. This “plateau” is similar to the metastable state – it’s not the final destination, but it delays the descent.
So, in simple terms, metastable states are a special kind of excited state in atoms that are long-lived because of the rules of quantum mechanics. They’re important because they provide opportunities for various technologies and applications.
What is a metastable state in physics?
A metastable state is like a temporary pause in the journey to the most stable state. It’s an excited state, meaning the system has more energy than it does in its ground state (the most stable state). But, unlike regular excited states that quickly decay to the ground state, metastable states hang around for a bit longer. They’re like those folks who love to linger at parties – they’re not going anywhere fast, but they’re not exactly at home either!
Think of it like this: imagine you’re balancing a pencil on its tip. It’s not stable, right? A tiny nudge and it’ll fall. But for a brief moment, it can stay upright – that’s like a metastable state. It’s not the most stable arrangement, but it’s possible.
Now, you might be wondering, “Why would anything hang around in a metastable state instead of just going to the ground state?” Well, it’s all about energy barriers. Just like the ball on the hill, there might be a little “bump” or energy barrier the system needs to overcome before it can reach the ground state. It’s like a tiny mountain the ball needs to climb over to get to the bottom of the hill. Sometimes, that metastable state can be a temporary holding pattern, a little pit stop on the way to the ultimate destination.
Let’s look at some examples:
Atoms: An atom can be in an excited state where an electron is in a higher energy level. This excited state might be metastable, meaning the electron hangs out in that higher energy level for a while before eventually falling back down to a lower energy level. This energy difference is sometimes released as light, which is how lasers work!
Nuclei: Nuclei can also be in metastable states, called isomers. These isomers are like slightly different versions of the same nucleus. They have the same number of protons and neutrons, but the arrangement of those particles can differ. A metastable isomer might last for minutes, hours, or even years before decaying to a more stable form.
Materials: Materials can also have metastable states. Think about a diamond. Diamonds are incredibly hard and sparkly because their carbon atoms are arranged in a very specific, tightly packed structure. But, there are other forms of carbon, like graphite, which is soft and flaky. Graphite is more stable than diamond, but diamond can exist because it’s metastable under the right conditions.
So, you can see that metastable states are pretty cool! They’re like temporary pauses in the journey to the most stable state. They can have all sorts of interesting effects and are important in many areas of physics and chemistry.
Which atomic energy levels are metastable?
So, some atomic energy levels are metastable, meaning that they’re excited states that are more stable than you’d expect. Rydberg atoms are a great example of these metastable excited atomic states.
Why are they more stable than other excited states? It all comes down to selection rules.
Electric dipole selection rules dictate which transitions between energy levels are allowed. These rules have to do with things like the change in the atom’s angular momentum.
Transitions from metastable energy levels are usually forbidden by these rules. This means that they’re less likely to happen, making the atom stay in that excited state for longer than normal.
Think of it like a ball sitting at the top of a hill. Normally, the ball would roll down, but if the hill’s surface is very smooth, it might just stay up there for a while.
That’s what’s happening with metastable energy levels. The atom is in an excited state, but the transition back to its ground state is less likely to happen, so it lingers there.
Here’s a little more about Rydberg atoms and why they’re metastable:
Rydberg atoms are atoms that have an electron in a high energy level, or what’s called a high principal quantum number (n). The higher the n value, the farther away the electron is from the nucleus, making the atom bigger. This also means that the electron is less tightly bound to the nucleus, so it’s easier to excite, and it can stay in that excited state for a long time.
But why are these high energy states metastable? Because the electron is far away from the nucleus, the interaction between the electron and the nucleus is weak. This makes the transition to lower energy levels less likely, making the excited state more stable.
So, while it’s true that the electron in a Rydberg atom eventually will fall back to a lower energy level and emit a photon, the transition can take a really long time, making the Rydberg atom incredibly useful in different areas of science, like studying how atoms interact with light or even for quantum computing.
How long do atoms stay excited in a metastable state?
You’re right, a metastable state is like a temporary pause button for an atom. It’s not the atom’s most stable form, but it’s not quite ready to jump back down to its ground state either.
Here’s the thing: atoms in a metastable state can stay excited for a surprisingly long time. We’re talking about a range of microseconds (10^-6 seconds) to milliseconds (10^-3 seconds). That might not sound like much, but in the atomic world, it’s a long time to be hanging out in an excited state!
To put it into perspective, imagine a ball perched precariously on the top of a hill. It’s not at its most stable position (the bottom of the hill), but it’s not going to roll down immediately either. It needs a little nudge, a little push to get it going. Similarly, atoms in a metastable state need a bit of energy to jump back down to their ground state.
So, why do atoms get stuck in these metastable states in the first place?
Think of it like this: the atom is like a little kid playing with a bouncy ball. They throw it up in the air, and it takes a while to come back down. That time it’s in the air, that’s kind of like the metastable state. It’s not the most stable position, but it takes a while for the ball to come back down to earth.
Now, let’s talk about what happens during this metastable period. It’s like the atom is holding its breath, everything about it is kind of “frozen” in that excited state. All the parameters associated with the atom’s state, like its energy level and its electron configuration, remain constant.
This “frozen” state also allows for a cool phenomenon called population inversion. Think of it like a crowded party where everyone’s crammed in one room, but there’s a whole other room that’s empty. Atoms in a metastable state are like the people in that crowded room. They don’t want to stay there, but they can’t get out easily. They need some extra energy to move to the “empty” room. This overcrowding of atoms in the metastable state is what makes lasers work!
Now, you might be wondering what makes an atom jump out of its metastable state? Well, there are a couple of things:
1. Stimulated emission: This is where an external photon (a little packet of light energy) comes along and kicks the atom out of its metastable state. This is what happens in lasers.
2. Spontaneous emission: Sometimes, an atom just decides to jump back down to its ground state on its own. It’s like the ball finally deciding to roll down the hill.
So there you have it! Metastable states are a fascinating way for atoms to temporarily store energy and can be used for some pretty cool applications!
See more new information: linksofstrathaven.com
Lifetime Of Electron In Metastable State: A Deeper Dive
Let’s dive into the fascinating world of metastable states and explore the lifetime of an electron within them. It’s a bit of a mind-bending topic, but I’ll break it down for you in a way that makes sense.
Imagine an electron in an atom. It usually hangs out in its ground state, which is like its comfy couch, the lowest energy level it can occupy. But sometimes, things get a little crazy, and the electron gets bumped up to a higher energy level. This is called an excited state.
Now, excited states are like those temporary “sugar highs” we get after eating a candy bar. The electron can’t stay there forever. It wants to chill back in its ground state. To do that, it needs to release the extra energy it absorbed.
There are two ways this can happen:
Fast and furious: The electron quickly drops back down to the ground state, releasing the energy as light. Think of it like a flash of light or a tiny explosion of energy. This process is called fluorescence.
Slow and steady: The electron gets stuck in a metastable state. Think of it like a stubborn friend who doesn’t want to leave the party. This state is still higher than the ground state, but it’s not quite as high as the initial excited state. The electron is “trapped” there for a bit, and it takes a little longer to release the energy. This is called phosphorescence.
So, what exactly is a metastable state? It’s like a little “detour” on the electron’s journey back to the ground state. It’s a forbidden state—meaning it’s not as readily accessible as other states. This means the electron has a hard time getting back down to the ground state.
The lifetime of an electron in a metastable state is how long it takes to get back to its ground state. This lifetime can vary significantly depending on the atom or molecule involved. Some metastable states might last for mere nanoseconds, while others can hang around for minutes, hours, or even days.
It’s like trying to get out of a maze: some paths are quick and easy, while others take a lot more time and effort. The lifetime of a metastable state is determined by the “maze” that the electron has to navigate.
Factors Influencing Metastable State Lifetime:
Several factors affect the lifetime of an electron in a metastable state:
Atomic or molecular structure: The type of atom or molecule plays a crucial role. The more complex the structure, the more likely there are pathways for the electron to relax.
Energy level differences: The larger the energy difference between the metastable state and the ground state, the longer the lifetime. Think of it like a steep hill: the harder it is to climb, the longer it takes to reach the top.
Environmental factors: Things like temperature, pressure, and the presence of other molecules can influence the lifetime of a metastable state.
Applications of Metastable States:
The concept of metastable states might seem abstract, but it has real-world applications, including:
Luminescence: The ability of certain materials to emit light after being exposed to light or other forms of energy is a direct consequence of metastable states. Think of glow-in-the-dark toys and street signs.
Lasers: Lasers rely on the population inversion of atoms, where more atoms are in an excited state than in the ground state. This is made possible by utilizing metastable states.
Atomic clocks: The highly accurate timing of atomic clocks is based on the precise transition frequencies between metastable states in specific atoms.
Measuring Metastable State Lifetime:
So how do we figure out how long an electron hangs out in a metastable state? We can use various techniques, including:
Time-resolved spectroscopy: This involves exciting the atoms or molecules and then measuring the decay of their fluorescence or phosphorescence over time. It’s like taking a snapshot of the electron’s journey back to the ground state.
Pump-probe spectroscopy: This technique uses two laser pulses to manipulate and probe the metastable state. It’s like sending a messenger to the metastable state to check on the electron.
FAQs
Q: What is the difference between fluorescence and phosphorescence?
A: Fluorescence happens when an electron quickly drops back to the ground state, releasing energy as light. It’s a quick flash. Phosphorescence happens when the electron gets stuck in a metastable state and takes longer to release the energy. This creates a more sustained glow.
Q: Are all excited states metastable?
A: No, not all excited states are metastable. Most excited states are short-lived and quickly decay to the ground state. Only those states that are “forbidden” for quick transitions are considered metastable.
Q: Why are metastable states called “forbidden”?
A: They’re called “forbidden” because the rules of quantum mechanics dictate that transitions between certain energy levels are less probable than others. It’s like a roadblock for the electron on its way back down to the ground state.
Q: Can metastable states be used for quantum computing?
A: Absolutely! Metastable states are crucial in quantum computing. The long lifetimes of certain metastable states allow for coherence—the ability of quantum systems to maintain their quantum properties over time. This is essential for developing quantum algorithms.
Q: How long can a metastable state last?
A: The lifetime of a metastable state can vary from nanoseconds to days depending on the atom, molecule, and environment. Some metastable states in specific atoms can have lifetimes of millions of years!
So, there you have it! The world of metastable states might seem complicated at first, but hopefully, I’ve shed some light on this fascinating topic. Now you know how electrons can get stuck in these “detours” and what makes them tick. These metastable states might be small, but their effects are far-reaching, impacting our understanding of light, time, and even the future of computing.
Metastable state | Phase transition, Kinetic energy, Equilibrium
Metastable state, in physics and chemistry, particular excited state of an atom, nucleus, or other system that has a longer lifetime than the ordinary excited states and that generally has a shorter lifetime than the lowest, often stable, energy state, called the ground state. Britannica
Metastable States – energy levels, forbidden
Solid-state gain media usually have a metastable electronic state as the upper laser level, and often some additional metastable states (energy levels). The upper-state lifetime , i.e. the lifetime of the upper laser RP Photonics
2.6: Resonant Tunneling, and Metastable States
Comparing Eqs. (152) and (154), we get a remarkably simple, parameter-independent formula \(^{32}\) \[\Delta E \cdot \tau=\hbar\] This energy-time uncertainty relation is certainly more general than our Physics LibreTexts
A time-domain phase diagram of metastable states in
The introduction of a time-domain axis in the phase diagram enables us to follow the evolution of metastable emergent states created by different phase transition mechanisms on different… Nature
Binding energies, lifetimes and implications of bulk
The metastable state collapses rapidly, which involves electron ejection and solvation dynamics, leading to the formation of a separated hydrated electron after approximately 500 fs (ref…. Nature
Chapter 3 Metastable State – Springer
Here, t1 is the supercooling time, t2 is the adiabatic jump time of the temperature, t3 is the isotherm freezing time, t1 + t2 is the existence time of the cooling metastable state, and Springer
A time-domain phase diagram of metastable states in a charge
Recent rapid progress in the time-domain investiga-tions of non-equilibrium phase transitions has led to the obser-vation of a variety of emergent transient and metastable Nature
Metastable States – Definition and Applications in Optics
Metastable state is an excited state of an atom or other system with a longer lifetime than the other excited states. However, it has a shorter lifetime than the stable ground state. AZoOptics
What Is Metastable State?
Electron Chemistry | Ground Vs Excited State
Where Do Electrons Get Their Everlasting Energy?
Metastable State In Laser || Metastable State
Laser
Basic Requirements Of A Laser System- Life Time , Meta Stable State || Engineering Physics ||
13.10 What Is A Meta Stable Nuclear State
Link to this article: lifetime of electron in metastable state.
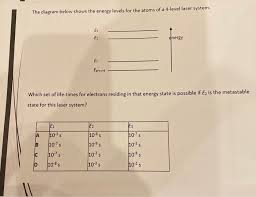
See more articles in the same category here: https://linksofstrathaven.com/how