What cells can fire action potentials?
Think of a neuron like a tiny electrical wire. It has a membrane that keeps the inside and outside separate. This membrane has tiny gates called ion channels that can open and close, allowing ions like sodium and potassium to pass through. When a neuron is stimulated, it causes some of these channels to open. This allows positively charged ions to rush into the cell, which changes the electrical charge inside the cell. This change in charge travels down the neuron like a wave, and that’s what we call an action potential.
Essentially, the neuron is using electrical signals to communicate with other cells. The action potential is how a neuron sends a message to another neuron, muscle, or gland.
Let’s break this down a little further:
1. Resting State: In its resting state, the neuron has a negative charge inside compared to the outside. This is because there are more negatively charged ions inside the cell and more positively charged ions outside.
2. Stimulation: When a neuron is stimulated, like by a neurotransmitter from another neuron, it causes some sodium channels to open.
3. Depolarization: The influx of sodium ions causes the inside of the neuron to become more positive. This change in charge is called depolarization.
4. Action Potential: Once the depolarization reaches a certain threshold, it triggers a chain reaction. More sodium channels open, and the electrical charge inside the neuron rapidly rises. This is the action potential.
5. Repolarization: After the peak of the action potential, potassium channels open, allowing potassium ions to flow out of the cell. This repolarizes the neuron, returning it to its negative charge inside.
6. Refractory Period: The neuron enters a brief refractory period where it is less likely to fire another action potential. This ensures that the signal travels in one direction down the neuron.
This whole process happens in a matter of milliseconds, and it’s what allows us to think, move, feel, and experience the world around us.
Which cells in the retina fire action potentials?
Ganglion cells are the only cells in the retina that fire action potentials. This is crucial because action potentials are electrical signals that travel along nerve fibers, carrying information from the retina to the brain.
Think of it this way: Ganglion cells are like the messengers of the eye, sending coded messages about what we see to the brain. They’re the only cells in the retina that can actually send these signals.
Now, the ganglion cells fire under all lighting conditions, whether it’s bright sunlight or dim moonlight. However, the firing rate is what actually tells the brain about the intensity of the light. A higher firing rate means more light, and a lower firing rate means less light.
Imagine a light switch: You can flip it on and off, but the brightness of the light depends on how much you twist the knob. The ganglion cells are like the light switch, always “on” in some way, but the brightness they “signal” to the brain depends on their firing rate.
Here’s a more detailed explanation of the ganglion cell firing process:
Light hits the retina: When light enters the eye, it hits the back of the retina, where the photoreceptor cells (rods and cones) are located.
Photoreceptors convert light into electrical signals: The photoreceptor cells convert light energy into electrical signals.
Signals travel through the retinal network: These electrical signals then travel through a network of other retinal cells, including bipolar cells, amacrine cells, and horizontal cells.
Ganglion cells receive the final signal: Finally, the signal reaches the ganglion cells.
Ganglion cells fire action potentials: The ganglion cells then generate action potentials based on the strength of the signal they receive, encoding information about the intensity and other characteristics of the light.
This firing rate is a fundamental aspect of how we perceive the world around us. It allows us to see in both bright and dim light, distinguish between different colors, and detect movement. The ganglion cells are truly the key to our vision!
Can glial cells fire action potentials?
Glial cells don’t fire action potentials, the electrical signals that neurons use to communicate with each other. This has led to a misunderstanding about their role in the past, but scientists are discovering just how important glial cells are.
Take astrocytes, for example. These glial cells are found in the brain and spinal cord, and they play a crucial role in supporting neurons. They help to regulate the chemical environment around neurons, providing nutrients and removing waste products. But astrocytes do much more than just keep things tidy.
Recent research has revealed that astrocytes are actively involved in the process of communication between neurons. They can sense and respond to neurotransmitter release, and they even release their own signaling molecules. This means astrocytes can influence the strength and timing of synaptic transmission, effectively shaping the way our brains process information.
Astrocytes are not the only glial cells that are changing our understanding of brain function. Oligodendrocytes form the myelin sheath, a fatty substance that insulates neurons and allows them to transmit signals more quickly. Microglia act as the brain’s immune cells, protecting against infection and injury.
The more we learn about glial cells, the more we realize how crucial they are to brain function. They are not just supporting players; they are active participants in the complex symphony of the brain.
Can photoreceptors fire action potentials?
While photoreceptors are essential for vision, they don’t actually fire action potentials like many other neurons. Instead, they generate graded changes in their membrane potential in response to light. This means that the strength of the signal, how much the membrane potential changes, depends directly on the intensity of the light stimulus.
Think of it like a dimmer switch on a light. The dimmer switch controls the intensity of the light, just like the photoreceptor controls the strength of the signal.
So, how do photoreceptors transmit information if they don’t fire action potentials?
The answer lies in the way they release neurotransmitters. When light hits a photoreceptor, it triggers a series of biochemical events that ultimately lead to the release of neurotransmitters at the synapse. This release is directly proportional to the strength of the signal, meaning more light equals more neurotransmitter release. These neurotransmitters then bind to receptors on postsynaptic neurons, which are the next cells in the visual pathway.
The absence of action potentials in photoreceptors is important for several reasons:
Sensitivity: It allows for a high degree of sensitivity to light intensity. Since the signal is graded, photoreceptors can respond to even very faint light stimuli.
Temporal Resolution: It allows for rapid changes in light intensity to be transmitted quickly. Since the signal is not dependent on the propagation of an action potential, it can change rapidly in response to changing light conditions.
Dynamic Range: The graded response allows photoreceptors to respond to a wide range of light intensities, from very dim to very bright.
Overall, the absence of action potentials in photoreceptors is a key feature that allows the retina to process visual information efficiently and effectively.
Do bipolar cells never fire action potentials?
Think of it like this: imagine you have a light switch. Most neurons are like a light switch that is either completely on or completely off. But bipolar cells are like a dimmer switch. They can be adjusted to different levels of brightness, but they don’t turn completely on or off.
This type of signal transmission in bipolar cells is important because it allows for more precise information about the light levels in the environment to be passed on to the next layer of neurons in the retina, which are called ganglion cells.
The fact that bipolar cells don’t use action potentials is not a limitation, but rather an adaptation that allows for a different and more efficient way of transmitting information. This difference is crucial for our visual system to function properly and for us to see the world around us.
What do amacrine cells do?
Rod photoreceptors are responsible for vision in low light conditions. They are much more sensitive to light than cone photoreceptors, which are responsible for color vision. However, the signals from rod photoreceptors are slow and can be difficult to interpret. This is where AII amacrine cells come in. They act as signal boosters for the rods, taking their slow, weak signals and speeding them up, making them easier for ganglion cells to receive.
Ganglion cells are the final output neurons of the retina. They receive signals from photoreceptors and other retinal neurons, including amacrine cells, and send this information to the brain via the optic nerve.
Think of the AII amacrine cell as a translator, taking the slow, quiet language of the rods and turning it into something more understandable for the ganglion cells. This allows for efficient transmission of visual information, especially in low light conditions.
AII amacrine cells are distributed throughout the retina, ensuring that they can receive signals from rods across the entire visual field. This helps to ensure that all visual information is processed properly.
Their importance to retinal function is further emphasized by their role in other aspects of vision. They contribute to various functions such as light adaptation, the ability of the eye to adjust to different levels of light, and motion detection, our ability to perceive movement.
While AII amacrine cells are a specialized type of amacrine cell, it is important to remember that the retina is a complex network of neurons, each contributing to the intricate process of visual perception. The AII amacrine cell is just one of many components that make this process possible.
What is the difference between horizontal and amacrine cells?
Horizontal cells connect to photoreceptor cells, the cells that detect light. They are involved in lateral inhibition, which helps to sharpen the edges of images and improve contrast. Amacrine cells connect to bipolar cells, which are the next layer of neurons in the retina, and they are involved in a wide range of functions.
Let’s break down the differences further:
Horizontal cells primarily deal with light intensity. They receive signals from photoreceptor cells and send feedback signals to modulate their activity. This feedback mechanism helps to regulate the response of photoreceptor cells to light, contributing to light adaptation and contrast enhancement.
Amacrine cells, on the other hand, have a more diverse range of functions, influencing the activity of bipolar cells and ganglion cells in various ways. They contribute to:
Temporal processing: Detecting changes in light intensity over time.
Movement detection: Recognizing the direction and speed of moving objects.
Color processing: Contributing to the perception of different colors.
Spatial processing: Enhancing the sharpness of images.
Amacrine cells are also known for their diverse morphologies (shapes) and connections. They can have dendritic fields, which are the branches of a neuron that receive signals, that span different parts of the retina, enabling them to integrate information from various locations. This allows them to influence the output of bipolar cells in a wide area.
The specialized connections and functions of amacrine cells make them critical for the efficient and accurate transmission of visual information from the retina to the brain. Their involvement in various aspects of visual processing, including temporal, spatial, and color processing, highlights their crucial role in our visual perception.
Do astrocytes fire action potentials?
Astrocytes, unlike neurons, don’t possess the same voltage-gated ion channels that are responsible for generating action potentials. These channels are essential for rapidly transmitting electrical signals along the neuron’s axon. Instead of relying on electrical impulses, astrocytes communicate using a different mechanism. They release signaling molecules called gliotransmitters, which influence the activity of surrounding neurons and other astrocytes.
This communication system is vital for maintaining the delicate balance within the brain. Astrocytes help regulate the concentration of neurotransmitters, ensuring efficient neuronal signaling. They also contribute to the formation of synapses, the junctions where neurons communicate with each other. Moreover, astrocytes play a crucial role in supporting the overall health of the brain by providing nutrients, removing waste products, and protecting neurons from damage.
While astrocytes don’t generate action potentials, they are crucial for brain function. Their ability to sense and respond to changes in their environment makes them active participants in brain activity, contributing to the intricate symphony of neuronal communication.
See more here: Which Cells In The Retina Fire Action Potentials? | Do Amacrine Cells Fire Action Potentials
See more new information: linksofstrathaven.com
Do Amacrine Cells Fire Action Potentials?
Do Amacrine Cells Fire Action Potentials?
So, you’re wondering if amacrine cells fire action potentials. The short answer is yes, but it’s a bit more complicated than that. Let’s break it down.
Amacrine cells are a type of interneuron found in the retina of the eye. They play a crucial role in visual processing by modulating the signals transmitted between photoreceptor cells and ganglion cells.
Now, you might be thinking, “What’s the big deal about action potentials?” Well, action potentials are the way neurons communicate with each other. They’re like electrical signals that travel down the axon of a neuron, carrying information from one part of the neuron to another.
So, when we say amacrine cells fire action potentials, we’re saying they’re actively participating in this communication network within the retina.
However, not all amacrine cells fire action potentials in the same way. There’s a wide variety of amacrine cell types, and each type has its own unique characteristics, including how they generate action potentials.
Types of Amacrine Cells
There are roughly 30 different types of amacrine cells identified in the mammalian retina, and they can be classified based on their morphology, neurotransmitter, connectivity, and physiological responses.
For example, some amacrine cells are cholinergic (use acetylcholine as their neurotransmitter), while others are glycinergic (use glycine). Some amacrine cells have dendrites that extend across large areas of the retina, while others have dendrites that are more localized.
This diversity in amacrine cell types means they play a variety of roles in visual processing, and their firing patterns can be very complex.
Action Potentials in Amacrine Cells: A Closer Look
Here’s the thing about amacrine cells and action potentials:
Some amacrine cells fire action potentials, while others do not. The ones that do fire action potentials often do so at a low frequency and in a very specific pattern.
Action potentials in amacrine cells are often graded. This means that the amplitude of the action potential can vary depending on the strength of the stimulus.
Amacrine cells often release neurotransmitters in a non-spiking manner. This means that they can release neurotransmitters without firing an action potential.
The Role of Amacrine Cells in Visual Processing
So, why do amacrine cells fire action potentials? How does this contribute to vision? It’s all about modulating the signals that flow through the retina.
Think of it like this: The photoreceptor cells are like the sensors of the eye. They pick up light and convert it into electrical signals. These signals then travel to the bipolar cells, which are like the intermediate processors. Finally, the ganglion cells are like the output neurons of the retina. They send signals to the brain, where they are interpreted as images.
Amacrine cells come in between the bipolar cells and ganglion cells, and they play a crucial role in refining the signals that travel to the brain.
Here are some examples of how amacrine cells contribute to vision:
Contrast enhancement: Amacrine cells can help to enhance the contrast of images by inhibiting signals from neighboring photoreceptor cells.
Motion detection: Some amacrine cells are specialized for detecting movement. They respond to changes in light intensity over time, which allows the brain to perceive objects in motion.
Color processing: Amacrine cells can also contribute to the perception of color. They receive input from different types of photoreceptor cells and help to integrate this information.
In essence, amacrine cells are critical players in the complex processing of visual information that occurs in the retina.
Understanding Action Potentials in Amacrine Cells
Here are some additional factors to consider about action potentials in amacrine cells:
Calcium channels: Action potential generation in amacrine cells is often dependent on calcium channels. These channels open in response to changes in membrane potential, allowing calcium ions to flow into the cell. The influx of calcium ions triggers the release of neurotransmitters.
Synaptic plasticity: The strength of the connections between amacrine cells and other retinal neurons can change over time. This process, known as synaptic plasticity, is thought to be important for learning and memory.
FAQs About Amacrine Cells and Action Potentials
1. What are the different types of amacrine cells?
As mentioned earlier, there are over 30 different types of amacrine cells, each with its unique characteristics and function. They can be classified based on factors like morphology, neurotransmitter, connectivity, and physiological responses.
2. How do amacrine cells contribute to vision?
Amacrine cells play a vital role in modulating the signals that travel through the retina. They help enhance contrast, detect movement, process color, and contribute to various other aspects of visual perception.
3. Why do some amacrine cells fire action potentials while others don’t?
The type of amacrine cell and its specific function influence whether or not it fires action potentials. Some amacrine cells rely on graded potentials and neurotransmitter release without spiking, while others utilize action potentials for communication.
4. How can we study the activity of amacrine cells?
Researchers use various techniques to study the activity of amacrine cells, including electrophysiology, optogenetics, and calcium imaging. These methods allow scientists to monitor the electrical activity and calcium dynamics of amacrine cells, providing insights into their role in visual processing.
5. What are the future directions of research on amacrine cells?
Ongoing research focuses on unraveling the intricate mechanisms of amacrine cell function, including their role in synaptic plasticity and how their dysfunction might contribute to various retinal diseases. Understanding these aspects holds potential for developing novel therapies for vision disorders.
There you have it! The world of amacrine cells is a fascinating one, and their role in visual processing is crucial. Even though their action potential patterns can be complex, they’re essential for our ability to see the world around us.
Narrow and wide field amacrine cells fire action potentials in
The pattern of action potentials evoked by current injections revealed two distinct classes of amacrine cells; those that responded with a single action potential (single-spiking Cambridge University Press & Assessment
Narrow and wide field amacrine cells fire action potentials in
Action potentials in amacrine cells are important for lateral propagation of signals across the inner retina, but it is unclear how many subclasses of amacrine cells contain PubMed
Amacrine Cells Can Intrinsically Fire Repetitive Action Potentials …
Transiently responding amacrine cells either remained transient or fired trains of action potentials when synaptic input was blocked, supporting both the intrinsic and the IOVS
Roles of Amacrine Cells – Webvision – NCBI Bookshelf
They are synaptically active in the inner plexiform layer (IPL) and serve to integrate, modulate, and interpose a temporal domain to the visual message presented National Center for Biotechnology Information
A17 Amacrine Cells and Olfactory Granule Cells: Parallel
The similarities between A17s and GCs cast into relief striking differences that may indicate distinct processing roles within their respective circuits: First, they employ National Center for Biotechnology Information
Visual System: The Eye – Introduction to Neuroscience
Photoreceptors do not fire action potentials; they respond to light changes with graded receptor potentials (depolarization or hyperpolarization). Despite this, the photoreceptors still release Open Textbook Publishing
Molecular identification of wide-field amacrine cells in mouse
B/K amacrine cells have long, unbranched processes and do not fire action potentials. To investigate the morphology and functional properties of single B/K eLife
Retinal processing: Amacrine cells keep it short and sweet
Amacrine cells are the first neurons in the visual system to fire action potentials, and also the first to generate transient responses. They send processes ScienceDirect
The fundamental plan of the retina | Nature Neuroscience
A simple concept of the retina’s function—lateral inhibition by horizontal and amacrine cells, a direct pathway mediated by bipolar cells—is part of the everyday Nature
2-Minute Neuroscience: The Retina
The Retina – Bipolar Cells, Horizontal Cells, And Photoreceptors
Receptive Fields And On/Off Center Bipolar Cells
The Schwann Cell And Action Potential
Developmental Mechanisms Shaping Direction Selective Circuits In The Retina
Action Potential – How Neurons Fire (Level 2, Intermediate)
Lights, Camera, Action Potentials!
Link to this article: do amacrine cells fire action potentials.
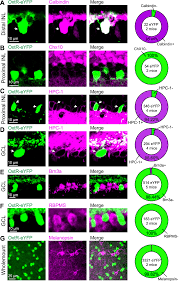
See more articles in the same category here: https://linksofstrathaven.com/how